Two-Pronged Strategy to Counter Brain Parasitism
Complex human disease is like an onion, requiring scientists to peel one layer at a time.
Figure 1. Cycle of Toxoplasma. Additional information about Toxoplasma is at Wikipedia and CDC. YouTube has several fun, educational clips: Toxoplasmosis - Plain and Simple, Toxoplasmosis and Mind Control - Plain and Simple.
Toxoplasma the brain parasite. Toxoplasma is the most successful parasite on Earth. It infects any warm-blooded animal. Its abilities to hide in the brain and alter behaviors of host organisms give fodder to science writers, who dub Toxo as the ‘puppet master’ and ‘mind bender’. Cloning itself everywhere, Toxoplasma has sex only in the intestine wall of feline species, also earning it popular media’s moniker ‘the cat parasite’.
The big debate. Debates have waged in the last decade on whether Toxoplasma causes a wide range of brain diseases. What is undisputed: this brain parasite infects at least one in three humans walking the Earth—that’s 2.5 billion Homo sapiens (Figure 1)!
My prediction in 2006 was this debate would not be settled for decades. Population-based studies of causality are crude tools for examining complex human diseases with multiple potential causes. Each one contributes a small fraction of the cascade. There is an urgent need for new thinking to narrow the mechanistic cause and experimentation. We need to view the complex problem more as an onion requiring us to peel one layer at a time.
The current dogma is illogical
I was first a protozoologist. I am forever amazed with the diversity, adaptability, and dynamics of simple organisms. These microscopic critters fullfill basic life needs like us, but as a single, nucleated cell. Protozoans are found everywhere. They adapt to harsh conditions, and when the timing is right, they can overtake an ecosystem such as the ‘red tide’ of dinoflagellates. Some evolved to live inside friendlier and self-contained ecosystems of human and animal.
A prevailing dogma since the discovery of Toxoplasma in 1908 never sat well with me. We are taught that this obligate protozoan living in our brains, albeit less active as cysts, is inconsequential. No proof, nor discussion, is needed. A single-cell organism camping out in your brain does nothing? Nothing good, or bad?
I am convinced Toxoplasma brain parasitism and diseases deserve similar attention as other global problems such as malaria and ‘neglected’ diseases on which Bill and Melinda Gates shine a bright spotlight.
Figure 2. My logic calls for a 2-prong strategy of killing the brain parasite and repairing the brain damages. Black arrow shows progress and red one indicates future opportunities for the research community.
Call to action. I returned to biomedical research for the last decade to ask three basic questions.
1). What gene network in the human brain is likely activated by Toxoplasma?
2). What diseases are correlated with brain parasitism?
3). What will we do about it?
My contributions. My goal is to facilitate a two-pronged strategy (Figure 2) for finding drugs to combat this human brain parasite. I use a 1-2 punch logic to kill the brain cysts and repair damaged neural networks.
I take an alternative path from other researchers (see BrainParasiter Journal). The base for the structural work was at the CSGID in Chicago, and later from my home in New Haven as BrainMicro LLC. I concentrate on shifting the research paradigm and building capacity to solve this global health crisis.
1). Decoding and linking gene networks modulated by Toxoplasma to human brain diseases.
2). Building a bedrock of crystallographic structure of proteins to guide drug design.
Prong I. Decode the gene networks linked to brain diseases.
Toxoplasma neuroscience and clinical studies. Drug targeting requires pinpointing the gene networks modulated by the parasite in the human brain. Yet, neurobiology of Toxoplasma is less studied. Clinical data from infected humans are sparse and clinical neurological studies are nonexistent. The last four decades of research investment have not produced a pre-clinical and clinical studies of potential drugs.
Human chronic diseases are a complex interplay of many genetic and environmental factors. I avoid the common approach of studying a singular gene/mechanism/pathway only because they are ‘trendy’ and ‘low hanging fruits’.
We need fresh and integrative thinking to identify and connect the multiple causes. I designed a new framework, collaborated with Dr. Rima McLeod, and dissected the correlations between chronic brain parasitism and disease. Dr. McLeod directs a small study group of children born with toxoplasmosis. Clinical samples collected since the 1980s provide the puzzle pieces needed to connect Toxo to human gene networks.
The result of a decade of work is now published with our collaborators. See ‘BrainMicro LLC’ for the international press coverage.
Toxoplasma Modulates Signature Pathways of Human Epilepsy, Neurodegeneration and Cancer. Ngô H.M. et al., 2017, Scientific Reports 7, Article number: 11496.
Shift the Paradigm
Figure 3. Four parts of Reconstruction. Susceptible genes are from a small cohort of individuals with toxoplasmosis. Biomarkers in brain serum are found for selected few with neurological problems. Neural stem cells are cultured from transected tissue of a hippocampus donated from surgeries. Neural stem cells are infected with Toxoplasma and assayed for changes in RNAs and proteins.
I changed the gene-environment paradigm to include a third part: ‘Development’. In this template, we have human susceptibility genes. The environmental factor is Toxoplasma infection. We used susceptibility genes of humans infected with Toxoplasma, all of whom developed various neuropathologies.
The overlap of gene and environment must alter a third piece of development, including plasticity in adults. Most brain diseases result from the abnormality of either process. We chose the primary culturing of human neural stem cells because they are the prime cell type and genetic program. These precursor cells regulate development and plasticity of the brain.
Reconstruction-Deconvolution Model. My ‘Recon-Decon’ model provides a likely snapshot of Toxoplasma effects in neurodevelopment and neuroplasticity. Brain infection reconstructs with four components of empirical data. The total dataset is then deconvoluted by system biological functions.
Reconstruction. Genetic input and readout are captured by susceptibility genetics and brain biomarkers of infected persons since birth. We assess potential parasitic effects on neurodevelopment and plasticity by determining the modulated RNAs (transcriptomes) and proteins (proteomes) of neuronal stem cells, which are cultured from individuals and infected in vitro.
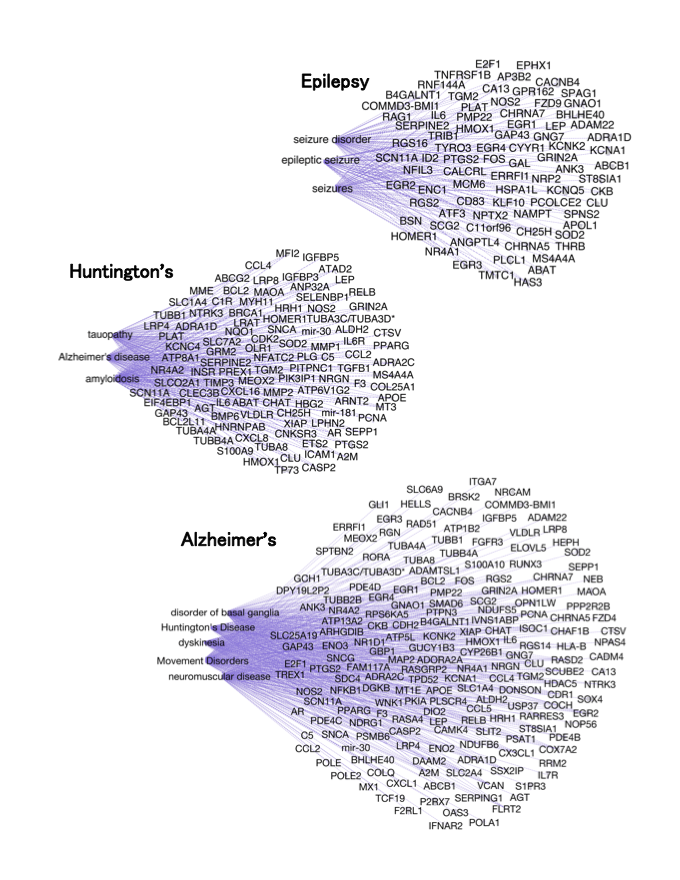
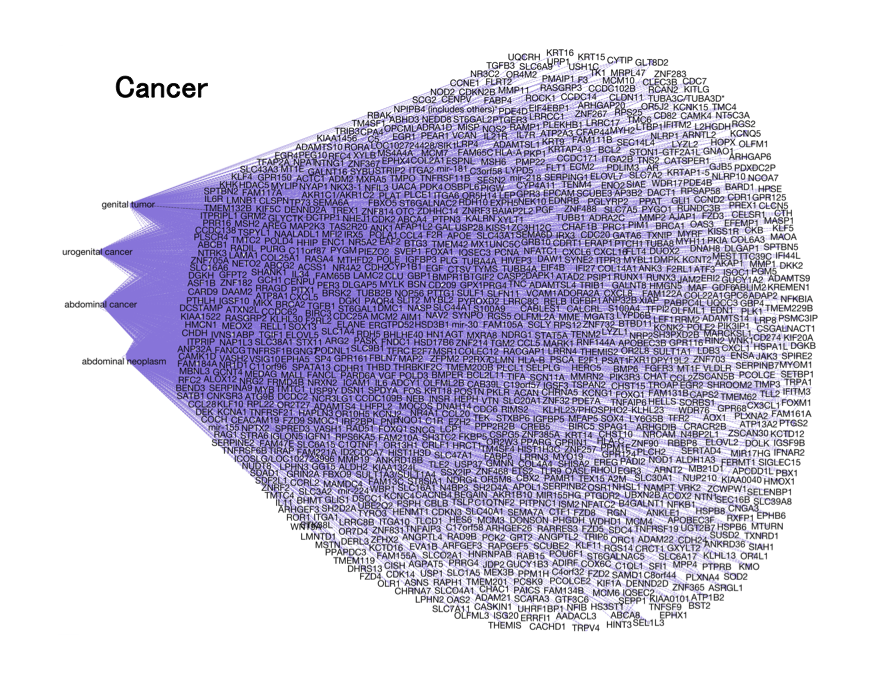
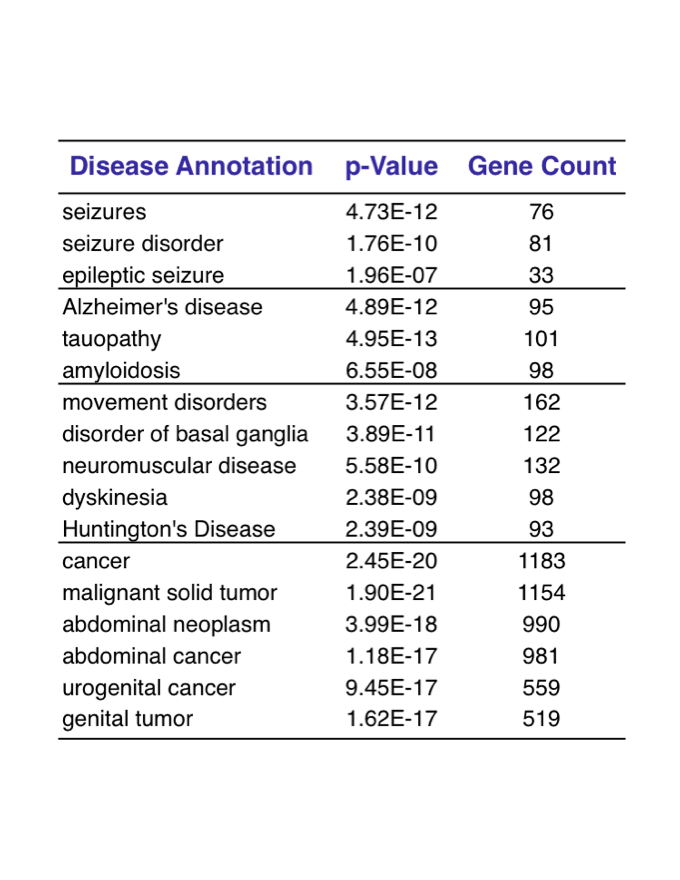
Deconvolution. Reconstructed brain infection (infectome,~1,500 genes) deconvolutes by three system approaches. “Orbital Deconvolution” includes a fifth part of upstream regulatory pathways showing interconnections between the four gene layers. “Cluster Deconvolution” identified seven visual clusters from a map of protein-protein interactions. The clusters detail processes affecting brain functions and circuitry, which includes alteration of olfaction. Parasite manipulation of smells in rodents and chimpanzees is well-documented.
The third approach of “Disease Deconvolution” is the most important. This analysis identifies correlation to gene networks associated with other brain diseases. They include: epilepsy (81 genes), Huntington's Disease and movement disorders (162 genes), Alzheimer’s disease (101 genes), and cancer (1,183 genes).
Figure 4. Disease Deconvolution. In this analysis, we correlate with significant statistics four diseases to Toxoplasma . Schizophrenia did not show up in this study with our prescribed specifications.
Why is this work important?
From the 20,000-25,000 protein-coding genes of the human genome, this strategy offers a direct route to generate a filtered human gene list. The gene networks identified using reconstructed brain infection most likely precipitate the neurological diseases afflicting Toxoplasma-infected humans. We peeled the first layer of the onion.
Figure 5. We complete the first step in pinpointing possible diseases correlating with brain parasitism. The probable gene networks are decoded. The next step is for our research community to associate these gene networks in human clinical studies.
These focused gene maps are logical starting points to confirm cause and disease effects of Toxoplasma brain parasitism with epidemiological, genetics, cellular and animal studies.
The disease gene networks can be refined with another deep-dive analysis to narrow down candidate genes for further studies. Such human genetic and epidemiological studies will increase the association ratio between candidate genes and a specific disease. For example, a clinical study of neural cancer patients can target these genes and networks. When we validate genes and functional pathology, the results give new direction to target our therapy.
The new paradigm and method model offer a logical systems roadmap to find leads in designing drugs and vaccines. This strategy with future fine-tuning is likely applicable to other human chronic diseases with complex multifactorial causes.
Complex human disease is like an onion, requiring scientists to peel with patience one layer at a time. We have peeled the first layer and are poised for the next mission.
Prong II. Drug leads to kill the brain parasite
Drug discovery and development. We discover drugs and therapies by two approaches, cellular or structural based. The choice fluctuates by decade in popularity. Cellular approach is in vogue by exploring new breakthrough techniques such as RNA interference and CRISPR gene editing. These newer technologies need decades of research to fulfill the promise from bench to bedside. The structure-based path has a long history with mixed results. Its advantages are high capacity and a wealth of experts and information on past failures and successes.
Figure 6. A partnership with Dr. Wayne F. Anderson (Former Director of CSGID) generated twelve enzyme structures that are potential druggable leads. Three collaborations produced publications as described below.
Drug search by protein structure for Toxoplasma was poor from my assessment in 2006. It remains so today with few structures solved. Those known are interesting biological features of the parasite, but most are poor candidates for drug targets.
My approach. My primary role in collaborating with CSGID was to build and coordinate the Structural Genomics Program for Toxoplasma (left prong, Figure 2). I selected parasite proteins based on three criteria. They are of high value for (1) drug targeting, (2) biological interests, and (3) ease of producing protein crystals. My secondary goal was to complement cellular and molecular researchers. We complemented their research with protein structures to advance their investigations in structure and function. I examined every Toxoplasma papers published and made the decisions from a whole perspective. We developed a global network of collaborators.
The progress. We completed three phases of the program that involved over 70 international research laboratories. Our team submitted over 300 proteins into the US structural genomics pipeline for potential works. This short 4-year period produced modest results with crystallographic structures of 12 Toxoplasma enzymes. They are deposited in the Protein Data Bank (see Drug Discovery). This contribution brings the total to only ~60 Toxoplasma proteins with their structure solved from the ~8,000 proteins annotated from the parasite genome.
But all twelve structures are small, druggable enzymes! They can serve in studying parasite biology and as leads for therapeutic intervention.
I packaged these projects for the global research community to use (see BrainParasiter Journal, Entry 7).
The joint efforts with our talented collaborators produced publications from three Toxoplasma enzyme structures.
Project 1
Membrane skeletal association and post-translational allosteric regulation of Toxoplasma gondii GAPDH1. Dubey R., B.L. Staker, I.T. Foe, M. Bogyo, P.J. Myler, Ngô H.M.* and M.J. Gubbels*. 2017. Mol Microbiol. 103:618-634. *Corresponding author.
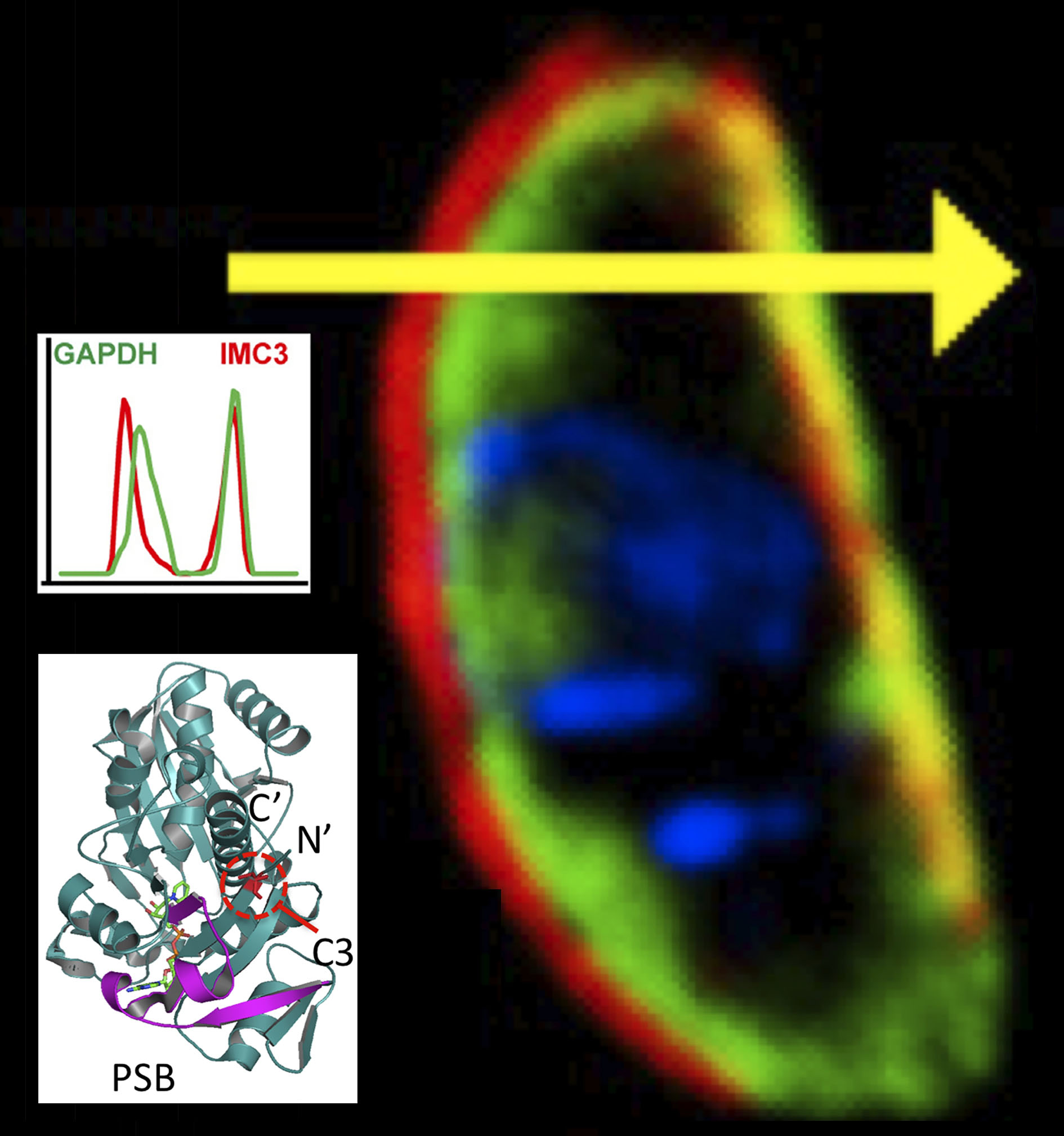
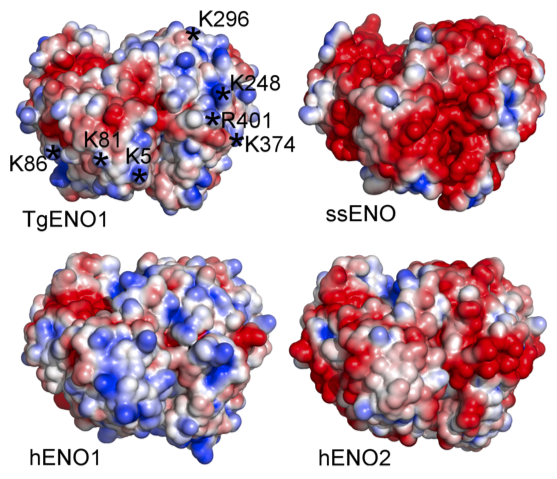
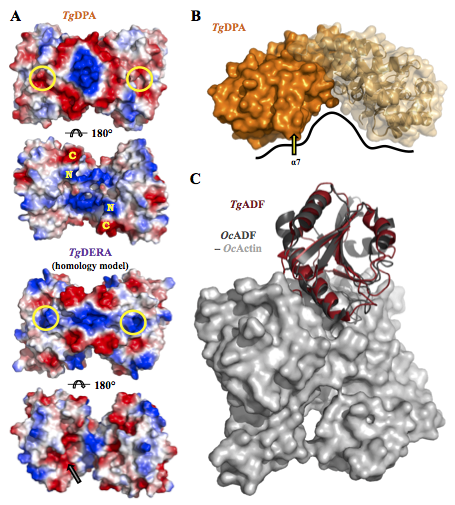
The manuscript is a collaboration with Dr. Marc-Jan Gubbels’ laboratory in Boston and Seattle Structural Genomic Center (SSGCID) of a ‘moonlighting’ enzyme.
When Toxoplasma liberates from the host cell, GAPDH1 protein in the cytoplasm binds to its unique membrane skeleton (cortex, Figure 4). Functional dissection of GAPDH1 decouples glycolytic role and cortical translocation. Glycolysis is essential, but cortical translocation is not.
We discover that N-terminal palmitoylation mediates GAPDH1 attachment to the membrane skeleton. Knockdowns of GAPDH1 expression and disruption of catalytic site confirm the essential role of GAPDH1 for replication in the host cell. We discovered phosphorylation of the S-loop of the protein as a novel regulator of GAPDH enzymatic activity. The dynamicity of S-loops is likely to be important for cofactor binding and allosteric activation of the enzyme.
Figure 7. Three Structure-Function Studies of Toxoplasma Enzymes.
We conclude that post-translational modifications (phosphorylation, palmitoylation) decouple the functions of GAPDH1 in glycolysis and cortical translocation in the cycle of the parasite liberating from the host cell. This publication provides a model for examining structure-function of Toxoplasma and other parasite proteins. Drugs designed to block GAPDH1 function can alter its metabolism and contribute to starving the parasite.
Project 2
The structure of bradyzoite-specific enolase from Toxoplasma gondii reveals insights into its dual cytoplasmic and nuclear functions. Ruan J., Mouveaux T., Light S.H., Minasov G., Anderson W.F., Tomavo S.* and Ngô H.M.*, 2015, Acta Crystallogr. D Biol. Crystallogr. 71:417-26. *Corresponding author.
The second manuscript is a collaboration between Chicago CSGID and Dr. Stan Tomavo’s laboratory in France. Dr. Tomavo described the enolases in Toxoplasma, serving dual functions as glycolytic enzyme and transcriptional regulator.
We examined for the first time the structure-function of a transcriptional regulator of Toxoplasma brain cysts (TgENO1, enolase 1) by combining crystallographic structure, transcriptional assay, and chromatin precipitation. Similar to a human oncogene (MBP1/hENO1, c-Myc promoter binding protein 1) that plays a role in transcriptional regulation, TgENO1 coordinates gene regulation in the development of brain cysts.
Enolase 1 provides a high value lead in the design of drugs against Toxoplasma brain cysts by blocking its bradyzoite gene transcription.
Project 3
Structural and functional divergence of the aldolase fold in Toxoplasma gondii. Tonkin M.L., Halavaty A.S., Ramaswamy R., Ruan J., Igarashi M., Ngô H.M., Boulanger M.J., 2015, J. Mol. Biol. 427:840-52.
The third manuscript is a collaboration set up between Chicago CSGID, Dr. Makoto Igarashi in Japan and Dr. Martin Boulanger in British Columbia to examine what was the first protein structure solved for Toxoplasma brain cysts.
Dr. Boulanger compares and contrasts the aldolase TIM barrel fold in two proteins that are expressed either in tachyzoite or bradyzoite stages. Tachyzoite aldolase (TgALD1) and bradyzoite pseudoaldolase (TgDPA) exhibit structural mimicry. Typical TgALD1 functions in glycolysis. TgDPA is restructured in its protein fold and it is inactive as an enzyme.
TgDPA plays a likely role in polymerizing the actin cytoskeleton during brain cyst dormancy. If validated, disrupting this protein may prevent the formation and lysis of Toxoplasma cysts in the brain.